Methane is a well-known and prominent greenhouse gas pollutant, contributing to the worsening effects of climate change on the global community. Such a pressing issue has led many in the scientific community to investigate potential solutions, with one of the most notable being the conversion of methane to value-added chemicals. These include syngas (a gaseous mixture of carbon monoxide and hydrogen gas, often used for synthesis or as a fuel) and compounds used for producing liquid fuel, like methanol. Generally, this conversion achieved through processes such as steam reforming, dry reforming, or autothermal reforming—all of which require considerable thermal input. To combat this obstacle, many have focused their attention on photocatalysis, an approach that does not require such excessive amounts of energy input.
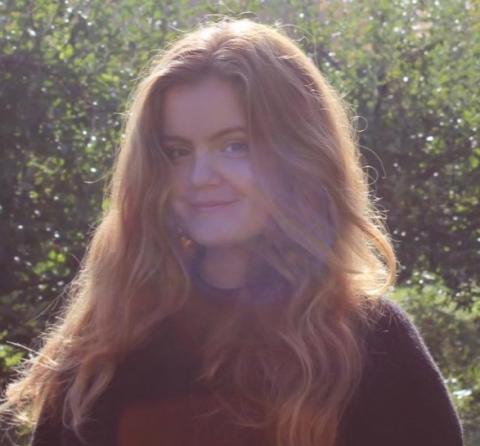
Isabelle Mann
This past summer, I received a Summer Undergraduate Research Fellowship (SURF) from the Hamel Center for Undergraduate Research to study the effectiveness of heteropoly acid (HPA) catalysts for the conversion of methane (CH4) via photocatalysis. Photocatalysis harnesses energy from light to drive chemical reactions forward. For this energy to be implemented into a given chemical reaction, a photocatalyst (a species displaying photochemical behavior) must be present. Semiconductors, such as titanium dioxide (TiO2), are often implemented as catalysts due to their exhibited photochemical behavior.
In photocatalysis, the photocatalyst receives the emitted photons from the light source, which then causes an electron-hole pair to form. These pairs act as charge carriers, speeding up the reaction mechanism. This generated pair is essential for photocatalytic activity, meaning the choice of photocatalyst is of the utmost importance.
Electron-hole pairs have a strong tendency to recombine shortly after generation. This recombination process significantly hinders the efficiency of the photocatalytic reaction. Therefore, in parallel with semiconductors, metallic species (such as nickel) are often implemented to decrease the rate of recombination. Metallic species are known to increase efficiency in photocatalytic reactions because of their ability to absorb the holes generated during the photocatalytic process, meaning recombination with electrons occurs less often.
In recent studies, it has been determined that HPA exhibits photochemical behavior similar to TiO2. However, HPA can absorb light in the UV range—a characteristic lacking in most semiconductors, including TiO2. Thus, the combination of the two species results in a higher absorbance spectrum of the photocatalyst, leading to more electron-hole pair generation. This increase in photoactivity, in theory, can then increase conversion rates.
The main goal of my project was to combine the photochemical behaviors of TiO2 and HPA, as well as nickel (Ni) as the added metallic species, in order to achieve photocatalytic methane conversion. The objectives were to understand how the ratios of Ni, HPA, and TiO2 contribute to methane conversion and selectivity, as well as what operating conditions—including the intensity of light, the amount of catalyst used, and the total run time of the reaction—were optimal for the reaction.
Photocatalyst Synthesis
To achieve these objectives, I synthesized Ni-HPA-TiO2 photocatalysts and conducted photoactivity testing. To create the photocatalyst base, I combined titanium (IV) isopropoxide and phosphotungstic acid hydrate (the TiO2 and HPA precursors, respectively) in a solution of 2-propanol. The mixture was then pH adjusted, heated, and calcined to form HPA-TiO2. If nickel was to be added to the photocatalyst, wet impregnation using nickel (II) nitrate hexahydrate was conducted. In total, I synthesized three successful catalysts: two Ni(10 wt.%)-HPA(10 wt.%)-TiO2 photocatalysts, and one HPA(10 wt.%)-TiO2 photocatalyst.
To conduct photoactivity tests, I loaded each synthesized catalyst onto a small amount of quartz wool, secured on the surface using a few drops of deionized water and vacuum dried. I then loaded the dried sample into the photoreactor and flushed it with helium gas for thirty minutes to remove any impurities and/or unwanted gases that may be present in the reactor. The lamp was then turned on, and a mixture of methane and helium gas was introduced to the system.
I used a gas chromatograph to measure and analyze gaseous species leaving the photoreactor. The collected data determined if the photoreactor apparatus was operating correctly, as well as if any products (methanol, carbon monoxide, hydrogen gas, etc.) were formed, which would indicate successful conversion of methane during the reaction.
Preliminary Findings
Though final results are forthcoming, we improved our catalyst synthesis methods this summer. Instead of mounting HPA onto TiO2 using the conventional commercial product, we synthesized HPA-TiO2 directly from the titanium precursor (titanium [IV] isopropoxide) and HPA. This approach is thought to improve HPA mounting on the TiO2 surface, creating a more uniform structure.
We also improved the overall photoactivity testing procedure. The addition of the quartz wool into the reaction chamber created a more stable support for the photocatalyst than the previously used filter paper. The paper was not durable enough to keep the particles from flying into the gas stream and was also at risk of combusting during irradiation. Quartz wool effectively prevents this. In total, we completed three successfulphotoactivity tests.
Though multiple tests were run at conditions thought to be appropriate for methane conversion, no product was measured in the gas chromatograph. This problem could not be resolved during my project timeframe. However, I am continuing to work on the project this fall in INCO 790. Dr. Yi and I are hopeful that increases in lamp power, photocatalyst amount, and irradiation time will lead to conversion.
For perspective, the average mass of my photocatalyst was approximately 1-5 mg, whereas similar research by other groups used around 30-50 mg of photocatalyst. Such a large difference in mass may be one of the factors preventing conversion from occurring in my experiment. I plan to review my other experimental conditions from this summer before attempting other activity tests. Assuming the change in operating conditions leads to conversion, I plan to vary the mass of nickel and HPA to gauge their relative effects on rates of methane conversion. For instance, I plan to determine whether the addition of nickel to HPA increases conversion rate, as well their ideal weight ratios. Given that I obtain sufficient data, I will present my research at the Undergraduate Research Conference in spring 2023.
Significance
This project is significant for two main reasons. First, greenhouse gas pollution is proven to be a leading contributor of climate change, which has had detrimental effects including fatal heat waves and extreme natural disasters. Therefore, using methane as a reagent for sustainable energy production effectively reduces the amount of methane in the atmosphere while also providing alternative energy sources to fossil fuels.
Second, the integration of photocatalysis into methane conversion eliminates the need for high energy input, which is both costly and environmentally harmful. Other conventional reforming methods, such as steam reforming, require high temperature/pressure inputs to overcome the bond energy of C-H bonds in methane. In contrast, the energy input in photocatalysis is obtained through light, allowing the reaction to occur in ambient conditions. Photocatalysis not only serves as a clean and efficient alternative to other reforming methods, but a more affordable one as well.
From my experience with SURF, I gained numerous valuable skills and lessons. Given the varying triumphs and challenges I encountered, I feel more confident in my abilities as a researcher. Furthermore, I feel prepared to tackle my educational and career goals following my graduation from UNH. Whether it be applying to graduate school or settling into a career in sustainable energy engineering, SURF helped me to get one step closer.
I would first like to thank Dr. Nan Yi for his guidance and unwavering support through both my REAP and SURF projects. Without his knowledge and leadership, these research endeavors would not have been possible. I would also like to thank my generous donors, Mr. Dana Hamel and Ms. Madelyn Ball, both of whom made my SURF experience possible. Finally, thank you to the Hamel Center for Undergraduate Research for both the REAP and SURF awards; with these research experiences, I have gained valuable skills and knowledge that will continue to push me towards my academic and career goals.
Author and Mentor Bios
Isabelle Mann is a junior from Warner, New Hampshire, pursuing a bachelor of science degree in chemical engineering. Isabelle is involved in both the Interdisciplinary Honors and Departmental Honors programs and is a member of the Alpha Chi Sigma fraternity. She was introduced to this research shortly after she finished a different project through the Research Experience and Apprenticeship Program (REAP), also under Dr. Nan Yi. With Isabelle’s experience in the catalysis lab and Dr. Yi’s mentorship, combined with her enthusiasm for renewable energy, an exciting Summer Undergraduate Research Fellowship (SURF) project was born. Through this, Isabelle learned how to synthesize catalysts for experiments and operate instruments necessary to analyze her findings. Overall, the satisfactory takeaways were being able to conduct the research process with efficiency and independence and delving further into the science behind using light as an energy source. Isabelle sometimes encountered frustration when she didn’t yield her desired results, which she now knows is the key to learning. Isabelle decided to submit her research to Inquiry so that she may share it with readers of other disciplines and communicate her ideas in a detailed manner. After graduating from UNH, Isabelle plans to attend graduate school and pursue a career in sustainable energy generation.
Nan Yi came to work at UNH in 2015 and is now an assistant professor in the Department of Chemical Engineering. He specializes in catalysis science, which is a great passion of his along with STEM outreach. Dr. Yi teaches core chemical engineering classes and electives that discuss how the various forms and uses of energy impact the environment. He has mentored thirty-eight undergraduate researchers, some of whom received grant awards from the Hamel Center for Undergraduate Research and others who were awardees of alternative fellowships and scholarships such as DOE-SULI and Goldwater. Six of his former students went to the top chemical engineering Ph.D. programs, including MIT, Tufts and Columbia. His first undergraduate research student to become an Inquiry author was Samuel Mercer, a chemical engineering major deeply interested in methane conversion catalysis, similar to Isabelle Mann. Dr. Yi mentored Isabelle through the Research Experience and Apprenticeship Program (REAP) followed by a Summer Undergraduate Research Fellowship (SURF), for which he transitioned from being an “active director” of Isabelle’s research to a “passive” mentor, giving Isabelle more freedom to explore unknowns. Although she met some setbacks with her experiments, Dr. Yi says that she always takes the initiative to seek guidance and advice. He is pleased with Isabelle’s growth as an independent undergraduate researcher through these experiences.
Copyright 2022, Isabelle Mann