Where do our thoughts and emotions come from? What exactly is consciousness? How do mental disorders arise, and how do you treat them? Many people ask themselves these profound questions and probably attempt to answer them by referring to the brain, the organ that shapes who we are and our perception of the world around us. However, not many people know that the field that focuses on these “brain” questions is neuroscience. I myself only discovered neuroscience during the last year of high school, when I was deciding what my major in college would be.

The author, Vladimir Tkachev, in the lab.
I became mesmerized by this multidisciplinary study of the nervous system and brain that involves research in physics, biology, psychology, engineering, computer science, chemistry, and genetics. Neuroscience research helps us gain more knowledge about the brain in general, but it also saves lives by uncovering the mechanisms behind neurological diseases and mental disorders, which a great number of people suffer from around the world. Carrying out neuroscience research in a lab is what excites me about this field the most.
I was introduced to hands-on neuroscience research when I joined the lab of Dr. Arturo Andrade in my junior year at the University of New Hampshire (UNH). I had learned a lot about neuroscience by taking courses during my first two years at UNH, but only when I started doing actual research in Dr. Andrade’s lab did I fully grasp what goes into brain research. I learned and performed many useful research methods and techniques, but the most important of these was electrophysiological recording, which is the recording of electrical activity in nerve cells.
I am publishing this commentary to introduce readers to one of the most common research techniques used in the field of neuroscience. I believe that you cannot truly understand a research field until you learn about the techniques the field regularly uses. By giving a step-by-step explanation of electrophysiology I hope to show readers that carrying out research is not as hard and intimidating as it may seem. I want to raise awareness that neuroscience research is actually fun and that any college student even slightly interested in this field should give it a try.
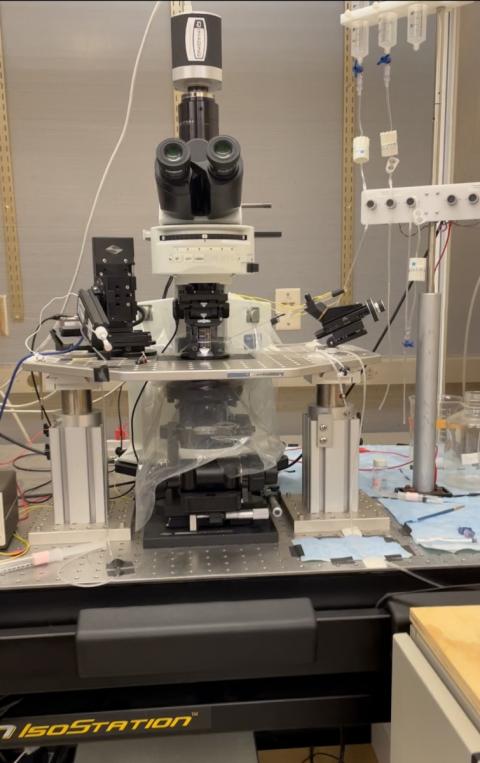
The electrophysiology rig that the author learned to use during his summer research.
Introducing Electrophysiology
The brain and nervous system work through electrical and chemical signaling. Brain cells send electrical signals and chemical messages to each other, enabling our brain to perform its functions. Electrophysiology is a branch of neuroscience that focuses on the electrical properties of brain cells, so electrophysiological recording is the most commonly used technique in every neuroscience lab. Learning this technique was crucial for me to understand the essence of neuroscience research.
I learned electrophysiological recording for a project called “Comparing the performance of glutamate sensors with conventional electrophysiology in vitro using hippocampal slices,” which I completed with the support of a 2021 Summer Undergraduate Research Fellowship (SURF). This project stemmed from a collaboration between Dr. Andrade and Dr. Edward Song, a professor of electrical and computer engineering who had designed a sensor that could potentially measure concentrations of glutamate, a chemical-signaling compound found in the brain. Dr. Andrade and I designed a project that could help Dr. Song test his sensor in a mouse brain. I felt excited to have my own neuroscience project to work on for the whole summer through SURF, as such opportunities are rare, especially for undergraduates. Dr. Andrade and I spent countless hours in the lab together, perfecting all the necessary techniques I needed to learn to complete this project. From the start of my work on the glutamate sensor project, my journey with electrophysiology began and my passion for this area of research started growing.
Glutamate’s Role in the Brain
Glutamate is a chemical-signaling compound that is essential for brain cell communication and is involved in many brain functions, including memory and learning. When a brain cell gets excited and undergoes a change in electrical potential, it releases glutamate, which in turn binds to a neighboring brain cell, inducing a change in potential in that cell. This mechanism on a grander scale involving millions of cells contributes to the brain’s ability to perform many of its functions. Glutamate dysregulations—problems with glutamate signaling—are involved in many neurological disorders; having a way to accurately measure concentrations of glutamate could provide researchers all over the world with a tool to quantify and study these dysregulations. Dr. Song’s glutamate sensor, which binds the glutamate itself, would provide a more accurate means of detecting glutamate concentrations than existing methods, most of which measure only the change in electrical current associated with glutamate release.
Preparing Mouse Brain Slices
My research goal was to measure glutamate in mouse brains, so the first thing I had to learn was how to extract a small, delicate mouse brain without damaging it. The brain has to be in perfect condition in order for electrophysiological experiments to work. After two weeks of practice, I was finally able to extract a brain in under a minute without damaging it. This timing is very important, as every second that the brain is not in an artificial cerebrospinal fluid solution can be lethal for the brain cells.
Next, I learned to make artificial oxygenated cerebrospinal fluid solutions that would keep the brain cells alive by providing a physiological environment for the slices. After only one week, I was able to make these solutions fast and without errors, and I was ready to move on to learning how to operate a vibratome apparatus.

Hippocampal mouse brain slices in a chamber filled with an artificial cerebrospinal fluid solution, which the author prepared
A vibratome slices tissue into thin segments (less than 1 mm) by sending frequent vibrations down a razor blade. To prepare the brain for the vibratome, I had to mount it on a cube of rigid gel and pour the oxygenated fluid solution around it to keep it viable. Then, after slicing, I had to immediately and carefully remove and transfer the slices with the help of a wide-tip pipette into separate chambers filled with the oxygenated fluid for preservation.
The next step in my training was learning how to operate the electrophysiological equipment. I learned to transfer a brain slice from its preservation chamber onto a tiny glass chamber in the electrophysiology rig. The slice was immobilized and a system was set up to send the oxygenated fluid through the slice. Learning this process took almost two weeks. The slices are very thin and can easily fall apart, so it took meticulous practice to transport and immobilize them without causing any damage. Moreover, setting up the perfusion system itself was a challenge because I had to find just the right rate of flow for the oxygenated fluid so that it did not leak out.
The trickiest part about setting up an electrophysiology experiment is inserting electrodes into the brain slice without puncturing it or damaging the cells. Typically, there are two electrodes, both thinner than a regular sewing needle. One is a stimulating electrode, which sends controlled electrical current, and the other is a recording electrode, which picks up electrical current and sends the recording to a computer for analysis. It was tedious to learn to move the electrodes exactly where I wanted them to be while also monitoring their location on a microscope. It took about five punctured slices for me to figure out just the right positioning. Mastering this skill was frustrating and exciting at the same time. I had to start over many times, but when I succeeded, it felt very rewarding.
Applying New Skills
For our experiment, we were interested in the hippocampus, a complex structure in the brain involved in memory storage and retrieval. Most memory loss disorders are associated with damage or dysregulation in the hippocampus. Most important for us, the hippocampus has high concentrations of glutamate, the neurotransmitter Dr. Song’s sensor was designed to measure. If this sensor proved effective, the way neuroscientists study neurological disorders could be revolutionized, because the sensor would be able to quantify glutamate concentrations more accurately than conventional methods do.
Two hippocampal regions were important for this experiment: CA1 and CA3. Essentially, region CA3 sends electrical signals to CA1, and CA1 receives them, undergoes a detectable change in voltage, and passes the signal farther down the brain. These two structures provide an electrical circuit in an area filled with glutamate, a perfect experimental model to test Dr. Song’s glutamate sensor.
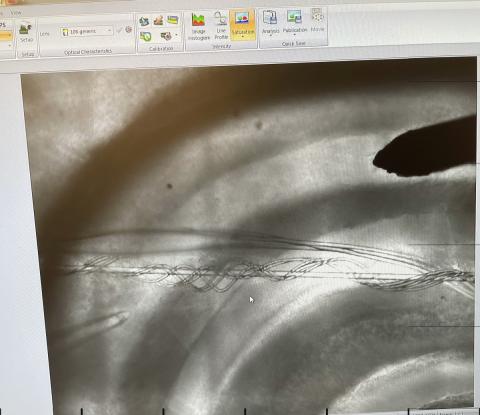
A microscope image displayed on a computer screen showing a mouse hippocampal brain slice with stimulus and recording electrodes inserted in it.
As part of the eventual sensor test, we would need to place a stimulating electrode in hippocampus region CA3 and a recording electrode in CA1. Reception of the electrical signal by the recording electrode would demonstrate that glutamate release was successful; however, it would not report the exact concentration of glutamate. We planned to place Dr. Song’s sensor, when it was ready for testing, in CA1 with the recording electrode and compare the sensor’s data with data from the recording electrode to see if the sensor could actually record glutamate release and also quantify it.
I learned to locate the CA1 and CA3 regions in a hippocampal brain slice, to carefully insert the appropriate electrodes, and to stimulate and record glutamate release. After a few tries of inserting electrodes into the slices and turning them on, I finally started seeing spikes on a graph on a computer, indicating that cell signaling was occurring and that glutamate was being released. That was a very rewarding feeling; everything I had been learning for the past three months finally amounted to producing healthy brain slices with functioning brain cells that could perform their signaling functions.
By the end of July my training was finalized, but I received sad news that Dr. Song’s sensor was not ready for testing yet, and the project was put on hold. Hearing this was quite upsetting, considering how much time I had spent training for this project. Nevertheless, I understood that situations like this are quite common in scientific research. The more complicated your project is, the more things there are that can take an unexpected turn, so this outcome was understandable.
Reflection
Even though I did not get to work with Dr. Song’s glutamate sensor, I now know arguably one of the most useful research techniques in neuroscience, and I will be able to apply this knowledge to future neuroscience projects in my career. I am ready to learn more intricate techniques, such as those so advanced that they deal with individual brain cells, where both stimulating and recording electrodes can be inserted into a single cell to study its properties. Through my experience I learned how neurotransmitters are studied in a lab setting, which is very useful, considering that the majority of neuroscience research touches upon aspects of neurotransmitters in one way or another.
The SURF research experience showed me that I was able to do difficult scientific research independently and that I could master a very complicated technique. Most important, participating in SURF shaped my plans for the future. With a good understanding of what rigorous research in neuroscience really is like, I have made the decision that I want to pursue a PhD in neuroscience at some point in my career in order to be able to conduct research in my own lab.
To conclude, I would like to mention that while the field of neuroscience is exciting because it helps us learn how our brains shape who we are as people and how we perceive our environment, most importantly neuroscience research saves lives. Neurological disorders such as Alzheimer’s disease, epilepsy, and Parkinson’s disease negatively affect the lives of millions of people. They are dreadful diseases because they do not just take a toll on your health but also affect your mind. Neuroscientists all over the globe work to figure out how these neurological diseases originate and to discover possible treatments for them. The more people are involved in this field, the greater our chances of defeating neurological disorders. And, as I have been fortunate to do, we might also learn one or two fun things about how our minds function along the way.
Special thanks to Dr. Arturo Andrade and Dr. Edward Song for guiding and supporting me throughout my journey with this project. The experience and knowledge you gave me will forever be with me, and I will use it to further my education and growth. Also, I want to thank the Hamel Center for Undergraduate Research and Mr. Dana Hamel for providing me with this rare opportunity to do advanced research with a 2021 Summer Undergraduate Research Fellowship (SURF) award.
Author and Mentor Bios
Vladimir Tkachev is a senior and an international student at the University of New Hampshire (UNH) from Vladivostok, Russia. In May 2022, he will graduate with a bachelor of science degree in neuroscience and behavior and a minor in biomedical sciences. He is a member of the National Honor Society in Neuroscience. Dr. Arturo Andrade, a former neuroscience professor at UNH and Vlad’s research mentor, assigned Vlad the research project that led him to the Summer Undergraduate Research Fellowship (SURF). This ten-week program was a brilliant opportunity for Vlad to gain hands-on experience in his field. Through the duration of this project last summer, Vlad learned more about nerve cells and the importance of using electrophysiology in his field. He found the process of research incredibly humbling, as it involved a great amount of patience, resilience, and persistence, especially when several things didn’t go as planned. For these ten weeks, Vlad felt fully devoted to the research study, which he enjoyed immensely and claims wouldn’t have been possible without Dr. Andrade. Vlad enjoys writing about science, and, thanks to Inquiry, the knowledge he shares will educate the general audience on a significant area of neuroscience. Vlad’s goal is to earn his PhD and become a researcher in neuroscience. He has no doubt of his passion for research and knows this is only the beginning of an exciting journey.
Arturo Andrade is a professor of brain science (research) at Brown University and specializes in neuroscience. He taught at the University of New Hampshire (UNH) from 2015 to 2021, when he moved to Brown. Dr. Andrade got involved in the project with neuroscience major Vladimir Tkachev through his UNH colleague Dr. Edward Song, an engineer studying ways to design novel sensing devices that accurately record concentrations of chemicals released in the brain. With Dr. Song interested in applying his sensing platforms in neuroscience research and Dr. Andrade being a neuroscientist, the collaboration couldn’t have been more perfect. Dr. Andrade previously mentored former UNH undergraduate researchers Sumeet Panesar and Zachary Tepper, co-authors in the 2017 issue of Inquiry. Dr. Andrade appreciates Vlad’s valuable contributions to the research team last summer.
Copyright 2022, Vladimir Tkachev